Developing a new drug has become a long and expensive process these days. Fewer drugs can get approved annually, and most of them fail in laboratories or preclinical phases. As science and technology advance, the approval process of a new drug has been becoming more complicated and needs more scientific studies to get to the clinic. On average, a new drug may need 10 – 12 years to be developed and this process may cost up to 2 – 3 billion dollars. We may wonder why developing a new drug is costly and how we can reduce it. To know where and how we should start, we should know that the main cost of developing a drug is spent in its clinical phase (usually two-thirds of the total cost). The traditional model to develop a drug involves in vitro cell studies and extensive animal testing. The next step would be the clinical testing, which takes two-thirds of the overall cost. This uneven cost distribution can show us something may be missing from the early stages to the clinical stage: a more complicated platform for studying a new drug. This new tool should be able to model human physiology more accurately. This led to the introduction of organ-on-a-chip technology by combing microfluidics and tissue engineering. If we want to define an organ-on-a-chip in simple words, we can say that an organ-on-a-chip is a miniaturized cell-culture tool with more complexity compared to the static cell-culture models. This advancement can be the integration of fluid microchannels, membranes for mimicking cell barriers, co-culture of different cells, mechanical or electrical stimulations, or the integration of biosensor for detecting a molecule of interest. Lung was the first organ to be modeled on a chip. The first breathing lung-on-a-chip presented by Huh et al. has attracted lots of attention from researchers as well as drug companies. Here, we review the current lung-on-a-chip models and their challenges and status to the market.
All the current lung-on-a-chip devices are aiming to recapitulate the complexity of the lungs to some extent to offer an advanced cell-culture model for drug discovery purposes. They usually consist of three components: (1) an air channel where epithelial cells can be grown and transferred later to air-liquid interface, (2) a “blood” channel that serves as the vascular part of the chip, and (3) a semi-permeable membrane to separate the air and fluidic channels. Together, all these new features can offer an in vitro model that has these advantages over more conventional in vitro models: (1)emulating the complexity of the lungs to some extent for instance by the integration of a stretchable membrane, (2)adding the respiratory breathing motions to the model either by stretching or the deflection of a thin membrane, (3) having “blood” and airflow in the apical and basal sides which can induce shear stress on cells and present a more realistic model than the other static in vitro models, and (4) the feasibility of using an extracellular matrix (ECM) based material as the membrane or added to the polymeric membrane. In some models, two sides of channels have been included to stimulate breathing by generating cyclic pneumatic pressure. This is helpful when the focus of the model is alveolar. Otherwise, a cyclic flow in the lower channel would be sufficient to mimic the physiological shear stress on cells. In addition, some biochemical factors such as proteins (collagen and fibronectin)or growth factors can be added to the chip (coated on both sides of the membrane) to recapitulate the cellular microenvironment.Polydimethylsiloxane(PDMS) is the most common material in the fabrication of these lung-on-a-chip devices since PDMS is optically transparent, biocompatible, easy to use, and flexible. Lung-on-a-chip devices aim to provide a tool for studying a drug. Besides, these devices can be used to simulate a lung disease such as inflammation, asthma, lung cancer, pulmonary fibrosis, or lung injuries and simulate the lung cells/tissue interactions at a “realistic” scale by controlling their microenvironment.
To begin with, we should mention the device designed and developed by Huh et al. in which mechanical strain was applied to stretch a porous PDMS membrane. They showed that the mechanical strain facilitated the uptake and transfer of nanoparticles by epithelial and endothelial. These results were confirmed by observing the similar effects in the whole mouse lung. The same research group later successfully modeled a human disease model-on-a-chip for pulmonary edema. This group led by DE Ingnercan be considered as one of the most dynamic teams in the field that has introduced various inventions not only to lung-on-a-chip devices but also to other organ-on-a-chip devices. In another study, they developed a small airway-on-a-chip and lined the airway with epithelial cells from patients suffering from chronic obstructive pulmonary disease. Using viral and bacterial infections, they could model the disease in their device. Moreover, they fabricated a microfluidic chip containing embedded electrodes for measuring trans-epithelial electrical resistance (TEER), which is used to monitor and quantify the integrity of cultured epithelial under statistic conditions. Later, they developed a lung alveolus-on-a-chip to model pulmonary thrombosis by co-culturing epithelium and endothelium. In another interesting work, KL Sellgren et al. designed a chip for culturing primary endothelial, epithelial, and lung fibroblasts to model the human airway. Polytetrafluoroethylene (PTFE) and polyester (PET) membranes were integrated between different layers of the chip. This work showed the feasibility of culturing primary airway epithelial cells with lung fibroblast and endothelium while providing appropriate compartmentalization in the chip. Such a triple co-culture with the capability of perfusion cannot be provided by the current commercial in vitro models. Other researchers also tried to integrate electrodes in a lung-on-a-chip device for monitoring the electrochemical and mechanical changing at the lung alveolar interface. Their device was also made of PDMS and mimicked the mechanical strain in alveolar by bulging a porous PDMS-based membrane. The advantage of this model is to create a 3D cyclic strain applied in all directions. An array of suspended gels was used instead of a porous membrane as a barrier between airway epithelial cells and airway smooth muscle cells in another work. There, the gel was a mixture of type I collagen and Matrigel which could enhance cell adhesion and growth. Xu et al.introduced an exciting approach to combine other organs in a lung-on-a-chip for studying lung cancer metastasis. This device had an upstream, lung, and three downstream organs that may be affected by lung cancer metastasis. These are only some of the works done in the field of lung-on-a-chip and more papers with details can be found in a recent review paper published in Biomicrofluics.
In the last few years, several organ-on-a-chip start-up companies have been founded, aiming to introduce a new platform for the drug discovery industry. Some of these companies offer devices at “body-on-chip” scales. Hesperos, Tissuse, CnBio, DRAPER are examples of these companies that are trying to provide a more complex model for assessing the pharmacokinetics and pharmacodynamic of new drugs on the human body than the conventional in vitro cell culture. Indeed, these models cannot completely mimic the complexity of the human body in the absorption, distributions, metabolism, and elimination of these new drugs but they are at least one step ahead of the available models. Some of the companies took another approach to construct an organ-on-a-chip that has a simulated tissue interface. Dr. Donald Ingber at Wyss Institute is one of the pioneers in this area and the founder of Emulate Inc. They offer a range of organ-on-a-chips including lung-on-a-chip or airway-on-a-chip. The strength of their technology is the ease of use for users as they developed several control modules. These modules can be used to control and monitor individual chips — another lung-on-a-chip company, Alveolix, founded by Dr. Olivier Guenatfor simulating the alveolar barrier. Not having vascular perfusion may be considered as a weakness, but it simplified the operation of the chip and would enable the users to operate multiple chips at the same time. Emulate and Alveolix are the most well-known companies in the area of lung-on-a-chip but there are more companies in the field. NORTIS, Quorum Technologies (Artery-on-a-chip Vessel), MIMETAS, SYnVIVO, 4DESIGN BIOSCIENCES, and AIM BIOTECH are good examples of these companies which have focused their efforts to bring some of the complexity of the human tissues to their in vitro models.
Regardless of all these advancements, there are still some issues that need to be addressed to introduce a more biomimetic model. For instance, the cell-to-liquid ratio should be improved to avoid the dilution of secreted proteins, metabolites, and factors. Fabricating smaller channels or growing cells in 3D may help to solve this problem. The thickness of membranes is typically around 10 µm which is much thicker than the basement membrane of blood vessels (300 – 400 nm). Molding hydrogel with integrated microchannels or 3D printing of hydrogels is a possible solution to tackle this challenge. The current models for alveolar can be improved by recapitulating the 3D shape of lungs air sacs. Instead of stretching the membrane that separates apical and basal sides, the membrane can be inflated by flowing air to the air channels.
To sum up, lung-on-a-chip and airway-on-a-chip are only one piece of the puzzle for developing a reliable platform in a drug discovery journey. As the future organs-on-a-chip or body-on-a-chip is supposed to be a comprehensive platform for testing a new drug, multiple chips should be combined to cover all aspects of human physiology. An interesting approach is to design a chip that mimics the human lungs at a smaller scale while having airways, bronchioles, and alveoli at the same time and show how various epithelial cells may interact with each other or which type is more prone to a specific disease or a drug. Realizing such a design can be very challenging based on the current technologies but 3D printing and bioprinting can bridge this gas and facilitate the progress toward a 3D lung model.
Enjoyed this article? Don’t forget to share.
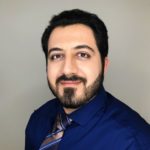
Mohammadhossein Dabaghi
Mohammadhossein Dabaghi is currently a postdoctoral fellow at the department of medicine at McMaster University. He completed his Ph.D. at McMaster University majoring in Biomedical Engineering and worked on new microfabrication technologies to develop an Artificial Placenta device for preterm neonates with respiratory failure. He earned a master’s degree in Biomedical/Chemical Engineering (2014), and a BS in Chemical Engineering from Sharif University of Technology, Iran (2012). His research interests focus on lung-on-a-chips, biosensors for organ-on-a-chips, and bioprinting.