Soft robotics is a growing field, especially for soft robots mimicking simple living organisms and the so-called Bio-bots1. These Bio-bots combine soft-materials (skeleton) with muscle cells, (muscle or motor) and can shine another light on the biomechanics of soft-bodied animals, such as the ray fish2 and the Octopus3. Macro-scale soft or hybrid gripers are emerging to substitute the standard rigid ones, which provides a manipulation tool for more fragile objects (example in Figure1)4,5,6.
In recent years, the advances in micro and nanotechnology and stimuli-responsive materials allowed the development of micro and nanoscale soft-robots. Why is so important to have a soft and relatively small robot? If we think about a tool to maneuver biological samples, being it for medical applications or simply for biological research, its mechanical properties (stiffness and elasticity, i.e., being soft or stiff and elastic or brittle) and overall dimensions should be comparable to properties of their targets. These miniaturized soft robots could potentially be used for medical operations, therapeutic or diagnostic, and drug delivery in a non-invasively way if they fulfill some important and challenging requirements.
To be able to access and navigate in certain areas of the human body these robots should be soft and small enough to avoid damaging tissues. Ideally, they would have a programmable shape, which would allow them to adapt continuously to the environment encountered. At the same time, these robots need to be soft enough to prevent failure when reaching the target areas and/or doing some in situ manipulation in medical treatments, if it is the case. The materials used should be biocompatible and easy to integrate functional materials, i.e., materials with a function of diagnostics or therapeutics7. As potential functionalities are thermal action for localized cancer treatment, hyperthermia, or cauterization of wounds; micro-gripping for non-invasive micro-biopsies and drug loading, for targeted drug delivery. In the case of microscale grippers/tweezers, if the stiffness of the microgripper could be reversibly and actively tuned would be a plus. It would be soft until reaching the target area, stiffened for actuation and return to its initial soft properties to be removed from the body7.
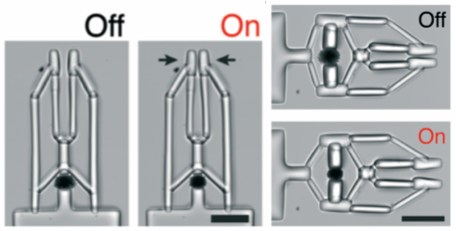
Figure 2. Left: Microgripper in the relaxed and actuated state. Right: Inverted microgripper mechanism in the relaxed and actuated state. Scale bars: 100μm8.
In this line of thinking, a group at EPFL, in Switzerland, developed different prototypes of microgrippers (Figure 2) that can be wirelessly actuated with light (see live actuation in the following video). These microgrippers could be potentially integrated with catheters for micro-biopsies, or simply used in the laboratory for microscale manipulation and transport of soft samples, such as cells, tissues, organoids or soft materials as is the case of hydrogels8,9.
Very briefly, the group used a microfluidic system to generate droplets with active nanoparticles, that subsequently will be cross-linked, resulting in a microsphere that can be actuated with light, i.e., upon light activation, these spheres can shrink, and swell when the light is switched off. This contracting/swelling property was used in analogy to the muscle contraction as a source of force. When connected to a skeleton, in this case, photopolymerizable hydrogel PEGDA, one can fabricate various tools that will be controlled with light, hence wirelessly.
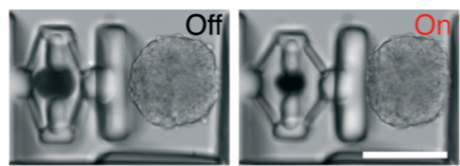
Figure 3. Compression of a spheroid of cells. Scale bar: 100μm8.
Besides the microgripper, the group also fabricated a soft-robotic compression device, Figure 3, to apply mechanical forces to 3D biological samples, being those, cell clusters, tissues or organoids; or even to measure their mechanical properties if a flexible probe is incorporated in the system8.
The fact that the skeleton of these structures is photopolymerizable, permits their fabrication directly inside lab-on-a-chip devices. This opens up the potential for the commercialization of new microfluidic devices for biomechanical studies and mechanical characterization of in vitro or ex-vivo biological samples8.
Final and briefly, the potential use of soft robots for drug delivery as an alternative to conventional systems is a very discussed topic. Target specific drug delivery still has a long way ahead before its commercial use, since: “Conventional drug delivery systems face several issues in medical applications, such as cyto/genotoxicity and off-targeting.”(Lucie Reinisová and colleagues)10 The use of soft-micro/nanorobots that can self-propel and navigate autonomously in fluids have a lot of potential. These small robots can hypothetically convert any external energy (chemical, ultrasounds, photo-electromagnetic fields) into motion. They can potentially have a higher cargo loading; precise targeting and on-demand release of the drugs, hence, reducing systemic side effects of highly toxic drugs10,11.
Featured image credit: Nebahat Yenihayat
1. Development of Miniaturized Walking Biological Machines; Vincent Chan, et.al; Scientific reports, 857, 2012
2. Phototactic guidance of a tissue-engineered soft-robotic ray; Sung-Jin Park, et.al; Science, vol 353, July 2016
3. An integrated design and fabrication strategy for entirely soft, autonomous robots; Michael Wehner et.al., Nature Letter, vol 536, Agust 2016
4. Programmable soft robotics based on nano- textured thermos-responsive actuators. Dong Jin Kang, et. Al., Nanoscale, 11, 2019
5. Soft Robotic grippers; Jun Shintake, et.al.; Advanced Materials Review; 30, 2018
6. Soft Robotics for Chemists; Filip Ilievski, et.al; Angew. Chem.; 50; 2011.
7. Miniature soft robots-road to the clinic; Metin Sitti; Nature reviews; 2018
8. Modular soft robotic microdevices for dexterous biomanipulation; Berna Özkale, et.al.; Lab on a Chip; 2019
9. Universal Soft Robotic Microgripper; Haiyan Jia, et.al; Small; 2018
10. Micro/nanomachines: what is needed for them to become real force in cancer therapy; Lucie Reinisová, et.al.; Nanosacle; 2019
11. Micro/Nanorobots at Work in Active Drug Delivery; Ming Luo; Advanced functional materials, 28, 2018
Enjoyed this article? Don’t forget to share.
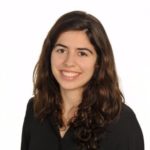
Raquel Parreira
Raquel Parreira is currently doing a Ph.D. in Bioengineering and Biotechnology at EPFL in Lausanne, Switzerland and she did her studies in the field of Biological Engineering at IST in Lisbon, Portugal. Her main focus is developing a variety of mechanically actuated systems for mechanobiology studies, for example, engineered biopolymers for in situ local mechanical stimulation and micro-soft robotic tools as the microgrippers and compressors recently published in Lab-on-a-Chip journal.