When commercializing a microfluidic technology, you start with a contraption put together from wires, tubing, pumps, power supplies, and microscopes, and you turn it into an instrument other labs can buy from a catalog-like a plate reader. Part of this journey is a transformation of your fabrication techniques. Here we will talk about the fabrication challenges when commercializing a microfluidic technology in an early stage startup; we will not look into other aspects of product development. Furthermore, we will mostly discuss the fabrication of microfluidic components and not other components like printed circuit boards.
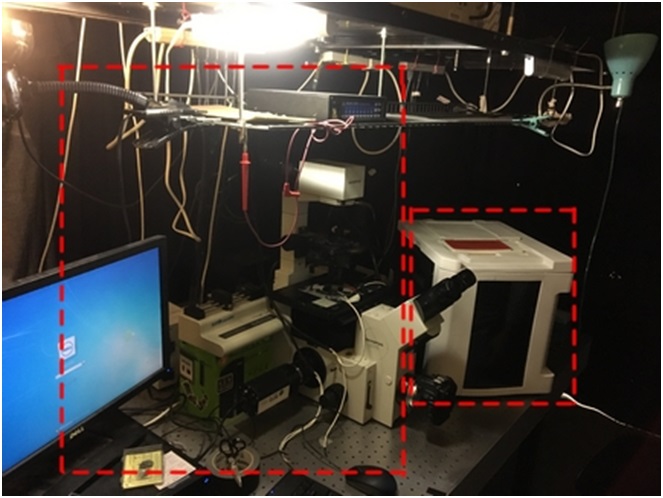
Left: Part of a microfluidic system in an academic lab, Right: A commercial prototype of the same system. (Image courtesy of CBio and Arizona State University)
In the noncommercial lab, no matter how final your design is, you always deal with prototypes: Made in very small numbers, subject to changes at any time, and often not a polished product. During commercialization, there are prototypes and commercial products. Most of what we discuss will apply to both, but the commercial product is the end goal, and the bar is higher for it than for the prototype.
A few new considerations pop up during commercialization:
- Requirements in production quality will be much more strict.
- Production volumes will go up.
- Cost restrictions will increase.
Quality:
In academia, I have heard this comment more than once: “Our fabrication is very reliable, we have about 90% success rate,” meaning 10% of their production was off-spec, but this was still a success. I have worked with wafers (PDMS casting molds) with four copies of a design where one was damaged, but I used it. My yield was just 75% of what it could have been. This is passable in academia because people fabricate for their own use, and don’t necessarily answer to an end user. Also, when only a few copies of a device are being fabricated, it may not be justified to fix all fabrication issues. With my wafer example above, if I anticipate a redesign, I wouldn’t remake a wafer since additional PDMS castings would be quicker and cheaper than a new wafer.
Obviously, you cannot tell your customer, “Don’t use channels 3 and 7, they are bad.” You also cannot afford to throw away 10% of your production run. The quality of the commercial product has to be the same as any other item you would buy for your lab commercially. Every device has to work, every time.
Volume:
Startups are not in the business of inventing great tech. They are just in business. Success will now be measured by how many instruments are sold. Most instruments are expected to sell in the hundreds to thousands of units, and any disposable parts are expected to sell a similar number for each unit of instrument sold. Fabrication methods available to an academic lab –or any research lab- are very inadequate. For example, making a batch of microfluidic chips in a cleanroom takes half a day to two days, and yields at best ~20 chips. Your startup may have 3D printing or CNC machining capabilities, but they will likely be limited to small batches of prototypes.
Most startups will likely need to contract a manufacturing company for their fabrication needs very early on.
Cost:
Money was scarce in the university lab, and it will be scarce in the startup. Money is always scarce, and the cost is always a big factor. In a startup, there is a new pressure that is seldom felt in academia. Being able to afford fabrication is no longer enough. Your cost has to be low enough that you can add a large margin on it and still sell at an acceptable price. I have recently used photolithography and wet chemistry techniques to produce a batch of microfluidic chip prototypes. They cost $357 per chip (and two days for a batch of 12). These chips were part of a disposable cartridge, and to be sold at my company’s target price, they needed to cost $1 or less! You may want to throw away money like this for your prototypes for testing your design, supplying your first customers, or other considerations.
Startups need fabrication methods that are more consistent, cheaper, and larger volume, which produce higher quality parts. That sounds unattainable, like the well-known triangle below, but industrial partners can make it happen.
Startups have two ways of having it all: Investors provide the financial muscle to contract the first large production runs, and sales to customers pay for subsequent ones.
What to do?
If your startup is part of an accelerator or an incubator, chances are some fabrication facilities will be available. Many university incubators focusing on tech companies provide access to the university’s cleanrooms at reduced rates. Others may have 3D printing or CNC cores, or even access to other fabrication technologies like roll-to-roll manufacturing. These facilities will be invaluable for prototypes, and not just for cost reasons. If your company has access to these, you have direct access to the staff, and your company’s personnel can go and fabricate your devices. More importantly, your turnaround times will not be tied to the schedule of an outside company, which usually take several weeks to fulfill orders.
Sooner or even sooner, you will need to outsource your fabrication. The key factors in choosing a vendor are:
- Cost (Depends on method and order size)
- Turnaround time (Typically several weeks)
- Type of fabrication method
- Smallest feature sizes, tolerances, cleanliness
- Range of services provided
Suppliers can provide services other than just making your part. They may take care of assembly, packaging, and testing as well. It’s also advantageous to source all parts of a subassembly from a single supplier. Parts from different suppliers are not guaranteed to fit together well, no matter how meticulous your CAD drawings.
When thinking about specs like smallest feature sizes, don’t forget about cleanliness, especially for machined parts. For example, when holes are drilled in glass parts with bits or lasers, debris (several microns in diameter) from the drilling falls on the part, which is a concern for narrow microfluidic channels. Conversely, when selecting glass or silicon substrates, wafer tolerances are seldom an issue because microelectronic and optic applications already have very strict tolerance requirements.
Injection molding: By far the most popular manufacturing method in the world, injection molding is best suited for large volumes –staples like syringes can have millions/month volumes. Injection molders will have larger minimum order sizes, likely at least 1,000. Small volumes are prohibited by the cost of the mold. The mold can cost anywhere from $5,000 to over $100k, but this cost is spread out over thousands of units. However, the slightest design change necessitates making a new mold, so this technique is better once designs are finalized. Injection molding typically has minimum feature sizes of ~20 microns, but companies such as Stratec and TechniColor have injection molding capabilities with submicron feature sizes; however, channel walls are significantly sloped in these cases. Injection molding is the most likely eventual route to large scale fabrication if it is suitable for your parts.
Machining: Various machining techniques allow parts to be made subtractively in a wide variety of ways. These techniques also have their setup costs which can be spread out over a large number of units. The complexity of design is a huge factor in the cost of machined parts. Unlike techniques like photolithography or wet etch, each individual feature in a machined part is formed separately. The number and complexity of features directly impacts fabrication time, and cost. A coverslip with a large number of inlet and outlet ports can be shockingly expensive because manufacturers have to charge machine time for each hole. “Organic” shapes and features that are not at the right angle to surfaces often cost extra. Your parts should be designed to be as efficient and cost saving as possible, and the best practices vary between techniques. The ability of a supplier to guide you in this regard should be a big indicator of their suitability for you1.
3D printing: 3D printing has become more popular and sophisticated in the past several years, and high volume production is now a reality. While a 3D printer sitting on your desk is a great way to prototype devices, note that these commonly available printers produce parts with textured surfaces, which may be unacceptable for your microfluidic devices. However, nanometer scale roughness can be achieved with commercially available 3D printing technology2. Setup costs are virtually nonexistent in 3D printing, which makes them cost-effective for very small production volumes, and also make it more amenable to design changes. 3D printing is also the most suited option for organic, irregular shapes. It should be considered strongly for prototype stages. Elveflow has an online guide for 3D printing technologies for microfluidics3.
Lithography and etching: These are likely to be very familiar methods to a microfluidics startup team, and your first prototypes are very likely to be made with these techniques. Outside suppliers can fabricate parts with these techniques at some volume, but these techniques tend to be expensive –they come into their own in the scales found in the electronics industry. However, all features are fabricated at once (e.g. all channels are etched simultaneously), so they can be an attractive alternative to machining, depending on the design4.
Note: Aytug Gencoglu has no conflict of interest regarding other companies mentioned or cited in this blog post. This blog post is not an endorsement of any companies or services.
1. Vaidya, N., Solgaard, O., 3D printed optics with nanometer scale surface roughness. Microsystems and Nanoengineering, 2018. v.4, article number: 18. DOI 10.1038/s41378-018-0015-4.
2. Elveflow, How to Choose a Microfluidic 3D Printer?. URL: https://www.elveflow.com/microfluidic-tutorials/soft-lithography-reviews-and-tutorials/how-to-choose-your-soft-lithography-instruments/microfluidic-3d-printer/
Last accessed: 03/01/2019.
3. Hudak, A., How to Cut CNC Machining Costs. Fictiv Hardware Guide, 2016. URL: https://www.fictiv.com/hwg/fabricate/how-to-cut-cnc-machining-costs Last accessed: 03/01/2019.
4. Gale, B. K., Jafek, A. R., Lambert, C. J., Goenner, B. L., Moghimifam, H., Nze, U. C., Kamarapu, S. K., A Review of Current Methods in Microfluidic Device Fabrication and Future Commercialization Prospects. Inventions, 2018. v.3(3) 60. DOI: 10.3390/inventions3030060.
For further reading:
5. Silverio, V., de Freitas, S. C., Microfabrication Techniques for Microfluidic Devices. Complex Fluid-Flows in Microfluidics, 1st ed., Chapter: 2, Publisher: Springer International Publishing AG, Editors: Francisco José Galindo-Rosales, pp.25-51. DOI: 10.1007/978-3-319-59593-1
Enjoyed this article? Don’t forget to share.
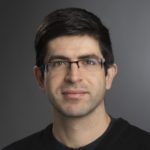
Aytug Gencoglu
Aytug Gencoglu has a decade of microfluidics experience, primarily in academic labs. He has previously published articles on electrode deterioration, pH changes in microfluidic channels, and dielectrophoresis, and developed microfluidics components for dielectrophoretic cell characterization.