A roundabout was recently constructed at an old 5-way intersection near my house. Pre-roundabout, it was a bit of a thrill. As a driver, I never really knew what to expect and would default to a “he who hesitates is lost” approach. As a pedestrian, a car came so close to mowing me down in one of the crosswalks that a police officer who was serendipitously on-the-scene decided to take a moment with the driver. Now the intersection is far less exciting. Traffic flows smoothly and safely as intended.
This story illustrates how I feel about much of the experimental work that I do and what I see others do in academic and clinical labs. There is an existing infrastructure sometimes far below the cutting edge of science and technology that “works” well enough to test your hypothesis and publish the paper you need for a better job where you can make others deal with the same inconvenient infrastructure. There are many logical reasons for sticking with what works, but, as in the case of the new roundabout, there is a point where superior technology becomes economical.
The tools used to develop therapies for diabetes, in all its forms, also need an upgrade. As with most biomedical research, two complementary platforms are generally used. On the one hand, cultured cells are an inexpensive resource for early-stage high-throughput studies. On the other hand, animals provide a complex, physiological environment to more accurately predict what is happening in the human body. However, the limitations of existing animal and cell culture models are not addressed by simply using both. Microfluidic platforms have natural advantages over both traditional cell culture and animal models. There are of course limitations here as well and these pain points are opportunities for commercial development. The advantages and current limitations of using microfluidic models of human tissues and organs are listed below to encourage their commercial development and adoption for diabetes research.
The Advantages We Need to Exploit
- Organ Modularity and Isolation: When experimenting on animals, it is often challenging to deduce the molecular mechanisms of disease traits due to the complexity of interactions between different organs, tissues, and cells. One can delete or overexpress a gene to demonstrate the respective necessity and sufficiency of that gene for the trait, but in which organ is the expression of that gene important? Tissue-specific genetic manipulation helps address this issue, but it remains challenging for diseases like diabetes where multiple genes operating in multiple organs contribute to pathogenesis. Moreover, many disease-associated genes and cell types are vital (i.e. lethal when deleted) necessitating alternative approaches. Engineered organ models can be connected to build systems such as the “body-on-a-chip” platforms discussed in a previous blog post1.Microfluidics aid in the logistical difficulty of combining multiple cell types, tissues, and organs as a synthetic vasculature to promote nutrient delivery and waste removal. Relevant organ, tissue, or cellular components can be added as needed and connected in a manner that enables sampling of the inputs and outputs for each component of the system.
- Controlled Human Genetic Background: Gene networks that are most relevant to diabetes such as those involved in immunity and metabolism are also the most evolutionarily distinct between mice, the predominant animal model for diabetes2, and humans3. In this regard, an experimental system with human cells is advantageous over mice. Multiple tissues derived from a single pluripotent stem cell line can be used for a completely isogenic model if desired. Alternatively, different genetic backgrounds can be combined to simulate transplantation, chimerism, or other experimental conditions. For example, combining five different organ models made from mutant and control cells from the same stem cell line is simpler than developing constructs for five separate genes each with distinct tissue-specific promoters. In addition, similar to the above point about vital genes, it is also conceivable that a tissue of interest lacks an established tissue-specific gene promoter.
- Data Quality, Automation and Cost Reduction: The temporal resolution of experimental data is often limited by the minimum sample volumes of well-based assays and the capacity of the human user. Microfluidics is naturally amenable to automated sample collection, reagent mixing, and measurement acquisition, reducing user error and manual effort. Microfluidics also have the inherent potential for cost efficiency due to the small amounts of reagents required4. Huge cost savings are possible if the supporting instrumentation is made to be simple and self-contained. By minimizing the time, error, effort, and expense of data collection, microfluidics simultaneously enable superior temporal resolution and data quality.
The Limitations We Need to Address
- Not quite in vivo…yet: Although genetic divergence favours a platform with a human genetic background for diabetes research, conventional cell culture falls woefully short of recapitulating important tissue-specific hallmarks of diabetes as well as the systemic nature of the disease. This is the primary criticism and limitation of cell culture and thus a major opportunity for microfluidic organ models. In my opinion, the key moving forward for diabetes research is less about making perfect and interconnected replicas of every organ in the body (which very well could take forever), and more about meeting a set of design criteria that cover the key features and phenotypes underlying specific hypotheses to be tested. The latter is achievable in our lifetime and can close the gap between patients and existing preclinical models.
- Not quite user-friendly …yet: A broadly accessible platform cannot become mainstream without industry’s help and probably its leadership, too. Academic labs simply cannot manufacture enough products with the necessary quality control for all the parties that stand to benefit by using it. Industry involvement will also be needed to achieve the right balance of standardization and flexibility. Utility for a broad range of applications will help the broad adoption of the technology. Finally, product designs need to integrate controls for tissue culture and functional readouts that allow operation without extensive training or expertise.
Conclusion
Diabetes research presents an exciting market opportunity for the development of microfluidic organ models and systems. The powerful advantages afforded by microfluidics are much needed to complement (and perhaps eventually replace) traditional cell culture and animal testing. If designed appropriately for non-expert users and with the right biological questions in mind, these platforms will be adopted and more importantly will help pave the new and improved road to a cure.
References
1. Organ On Chips: Questions To Address Before They Can Move Into Mainstream Applications. URL: https://ufluidix.com/circle/organ-on-chips-questions-to-address-before-they-can-move-into-mainstream-applications/
2. King A. The use of animal models in diabetes research. British Journal of Pharmacology. 166(3) (2012).
3. Yue F, Cheng Y, Breschi A, et al. A comparative encyclopedia of DNA elements in the mouse genome. Nature. 515(7527) (2014).
4. https://www.fluigent.com/microfluidic-expertise/what-is-microfluidic/microfluidic-definitions-and-advantages/
Enjoyed this article? Don’t forget to share.
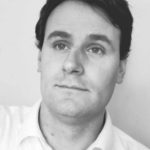
Benjamin D. Pope
Ben Pope works with a group of scientists and engineers who aim to cure diabetes. His current research leverages cellular engineering and genomics to elucidate the molecular bases of human nutrition. Originally trained in molecular biology, he identified DNA elements that define structural and functional chromosome units by both chromosome engineering in embryonic stem cells and computational analysis of genomic datasets. He has since cross-trained in bioengineering and developed a microfluidic chip for continuous sensing of insulin secreted by ex vivo human islets described in a previous blog post.