The lungs are the last organs to develop and mature before birth. A preterm baby, born earlier than 37 – 39 weeks of pregnancy, will have underdeveloped lungs and consequently will be under respiratory distress, struggling to breathe. As a result, these underdeveloped lungs are not able to produce surfactants, a slippery substance that keeps the air sacs open in inhalation, and easily collapse during exhalation. According to the World Health Organization, premature birth is the main reason of death in children aging less than 5 years and its rate is increasing all over the world. Every year 15 million premature births occur resulting in 1 million death each year due to complications of preterm birth. Even survivors may wind up with long-term complications in their adulthood.
Preterm (premature) babies with RDS (respiratory distress syndrome) require additional respiratory supports to let their lungs develop. Usually, mechanical ventilation is the first option to deal with RDS. If mechanical ventilation is not sufficient, another life support device, named extra-corporeal membrane oxygenation (ECMO) machine, will be used. In ECMO, the blood is pumped outside of the body and oxygenated by a gas exchange device called the blood oxygenator. Both methods are invasive and can cause severe long-term side-effect and complications. In mechanical ventilation, positive pressure applied in the lungs can seriously damage the tissue of the lungs. ECMO may be less destructive to the lungs but it has own issues, especially for those babies with extremely low birth-weight. Generally, ECMO devices need a high volume of blood to be primed (for instance around 40 mL for the device such as Quadrox-i Neonatal & Pediatric), however, a one-kilogram preterm baby has only about 100 mL of blood. As a result, donor blood from adults needs to be transfused to keep the whole system operational. Nonetheless, baby blood and adult blood have different physiology in which red blood cells (RBCs) of newborn babies have a higher affinity to oxygen compared to RBCs in adults. Additionally, surgery is required to get access to the large vessels and a pump is needed to keep blood flowing in the circuit which may damage RBCs and lead to hemolysis and thrombus formation.
Artificial Placenta as an Alternative Solution
The artificial placenta (AP) concept refers to a postnatal lung assist device (LAD) which is connected to a premature baby via the umbilical cord and can provide additional gas exchange besides the baby’s lung. The AP concept has been around for a long time back to 1961 when the first attempts were made by Callaghan et. al. However, there is still no commercial AP device in clinics due to inefficiency and incompatibility of available LADs for this purpose. An ideal AP device should be operable by a baby’s heart instead of an external pump while provides sufficient gas exchange in room air. Also, such a device should have very low priming volume to eliminate any need for donor blood transfusion and be filled only with saline solution. In addition, the volume of needed saline should not surpass 10 percent of the total blood of the baby’s body to avoid blood dilution. The first and main job of an AP device is to compensate for the loss in gas exchange caused by under-developed lungs. Also, some effort was made to expand AP application more and provide nutrition to premature babies and remove the metabolic waste at the same time.
Conventional blood oxygenators (used for ECMO) which are usually made from hollow fiber membranes are not suitable for artificial placenta application as they have high priming volume and need a pump for operation. A hollow fiber membrane (HFM) device is made by twisting bundles of hollow porous tubes (fibers) in a plastic-made shell. Usually, oxygen is introduced to these fibers and blood flows around these porous fibers where oxygen transfers to blood and carbon dioxide is released from the blood. Because of the nature of the hollow fiber bundle, the blood experience a non-physiological travel which may damage RBCs and initiate clotting. Also, there is a great potential of blood leakage through pores or contamination of the blood. An ECMO device is connected to the body in venous-artery or venous-venous configuration where a pump is needed to remove blood from the body and send it back to the ECMO device and vice versa.
It should be noted that an AP device is not going to operate in the same way that an ECMO machine works. In fact, an AP device is connected to a baby’s body in parallel to the blood circulatory system wherein the inlet of the device is attached to an artery and the outlet to a vein. This particular type of arterio-venous connection causes the blood entering the device has already been traveled through the baby’s lungs and be partially oxygenated. Under-developed lungs of a premature baby cannot fully saturate the blood with oxygen, whereby the oxygen saturation level may only be 10%–30% below full saturation. Therefore, an AP device only needs to raise the oxygen saturation level from ~ 70 % to 100 % which is higher than the amount of oxygen saturation level entering an ECMO device.
The Solution
Over the last decade, researchers have been using microfabrication technologies to overcome the shortcoming of HFMs and trying to introduce the next generation of blood oxygenators. Microfluidic blood oxygenators have shown promising improvement in gas exchange compared to convention ECMO devices over the last decade due to mimicking nature lungs by reducing the size of blood channels as small as capillaries in the lungs. Additionally, microfluidic blood oxygenators provide a more physiological flow path to the blood which is critical in designing an AP device for the long-term application.
Recently microfluidic blood oxygenators have been specifically designed to address the needs of the neonates and have been developed with low priming volume, low-pressure drop to operate pumpless, and capable of working in the ambient air. Rochow et. al.used microfluidic blood oxygenators to construct such a LAD for premature babies suffering from RDS. Their device had a low priming volume of 4.8 mL and could be successfully connected to the umbilical vessels of newborn piglets and perfused solely by the pressure differential provided by the animals’ heart. Although the gas exchange of this LAD was not sufficient enough but they showed that a pumpless LAD with low priming volume was not a fantasy anymore!
Challenges
Truly, the future belongs to blood oxygenators advancing microtechnologies owing to their unique design so much similar to nature lungs. However, they need to overcome some challenges to be able to compete with HFMs and replace them in the markets. The main challenges are listed below:
- Scaling up: the main and most important limitation of all microfluidic blood oxygenators is their small size which constrains their operating blood flow rate below 10 mL/min. However, an AP device may need to support a higher blood flow rate up to 60 mL/min. Different strategies have been used to address this issue such as increasing the size of the device or connecting several devices together to increase the total capacity. It seems like a combination of both should be used to reach the desired gas exchange.
- Hemocompatibility: although different approaches such as coating the surface of PDMS by antithrombin-heparin (ATH) or polyethylene glycol (PEG)have been used to improve the hemocompatibility of the blood-contact surfaces, more investigation is required to evaluate this coating reliability for a long-term condition as needed for an AP device.
- Access to large bore vessels: an AP device is supposed to be connected to a premature baby via the umbilical cord. The main challenge is the constriction of the umbilical vessels right away after birth. As a result, a catheter is required to be inserted into the vessels and keep the vessels open and let blood flow with minimum resistance to blood flow. As Peng al. explained, a special type of catheter should be used for getting access through the umbilical cord to be able to minimize the resistance to blood flow and provide the maximum possible blood flow rate for an AP device.
Enjoyed this article? Don’t forget to share.
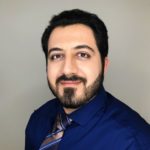
Mohammadhossein Dabaghi
Mohammadhossein Dabaghi is currently a postdoctoral fellow at the department of medicine at McMaster University. He completed his Ph.D. at McMaster University majoring in Biomedical Engineering and worked on new microfabrication technologies to develop an Artificial Placenta device for preterm neonates with respiratory failure. He earned a master’s degree in Biomedical/Chemical Engineering (2014), and a BS in Chemical Engineering from Sharif University of Technology, Iran (2012). His research interests focus on lung-on-a-chips, biosensors for organ-on-a-chips, and bioprinting.