When it comes to microfluidics or microfabrication, almost everyone thinks of PDMS or Poly(dimethylsiloxane). Since George Whitesides in 1998, for the first time introduced the use of PDMS in microfluidics, PDMS has become the primary material of choice, and it has been playing an essential role in microfluidics. This article reviews some of PDMS’s advantages and the role PDMS is playing in Microfluidics.
In general, PDMS belongs to the silicone family with some unique features which have turned PDMS to the most desirable material in microfluidics. Transparency, biocompatibility, flexibility (Young’s elastic modulus of ~ 1 – 3 MPa), high gas-permeability, low dielectric constant, low surface tension, and low solubility are some of PDMS characteristics.
The PDMS strength stems from its capability in soft lithography. First, PDMS base monomer is thoroughly mixed by its curing agent, then this prepolymer is degassed to remove all air bubbles, and finally, it is ready to be poured on the mold. The mold can be made by conventional methods such as photolithography or newer technologies such as 3D printing. Regardless of the mold type, PDMS can replicate features on the mold from macroscale to nanoscale. After curing and peeling off the PDMS replica from the mold, the PDMS part should be sealed by a flat surface. Here, another advantage of PDMS fabrication comes to play. There are a variety of techniques to seal a PDMS replica such as conformal contact, physical bonding, vacuum bonding, oxygen plasma bonding, corona surface activation, flame bonding, wet-bonding, adhesive bonding, and to name a few. Some of these methods are reversible, and some are irreversible which can be chosen based on applications. In most of these techniques, there is no need to use a chemical or solvent to achieve the sealing. The chemical- or solvent-free bonding of PDMS to PDMS substrates or other substrates eliminates any chance of chemical contamination to tested samples.
PDMS soft lithography allows researchers to fabricate devices with multilayers of PDMS. This process is called “sandwiching,” meaning that several layers of PDMS replica can be stacked on top of each other to build a more complex geometry. In sandwiching, other components such as membranes (porous or non-porous) can be added between layers to create the desired device. There are different ways to bond these membranes to PDMS: (1) membranes first can be treated by a silane molecule (such as 3-aminopropyltriethoxysilane), and then both treated membrane and PDMS are exposed to oxygen plasma and be bonded, (2) some adhesive (double-sided tapes or adhesives) may be used to attach membranes to PDMS if no high pressure is sought, and (3) silicon dioxide (SiO2) can be coated on a membrane by sputtering and be bonded to PDMS using oxygen plasma.
There are a lot of strategies to tailor the bulk or surface properties of PDMS. This allows researchers to modify their PDMS devices based on their needs. For tailoring bulk properties of PDMS, these methods can be used: (1) changing the ratio of base and curing agent, (2) playing with curing conditions (temperature and time), (3) adding other molecules to PDMS, and (4) adding fillers such as SiO2 to PDMS. Playing with these parameters can impact the bulk properties of PDMS such as elasticity, transparency, photothermal effect, refractive index, electrical conductivity, and to name a few. The review paper which was written by P. Wolf et al., comprehensively reviews all different techniques for PDMS bulk or surface modification. In most scenarios, surface modification of PDMS is the area of interest in microfluidics. The most common way is to use oxygen or air plasma, corona discharge, and ultraviolet light or ozone exposure to introduce silanol (Si-OH) groups to the surface of PDMS at the cost of methyl groups (Si–CH3). This results in forming a thin layer of few nanometers on the surface (it may contain some cracks as well) and decreasing water contact angle to lower than 5°. When a long-lasting surface modification strategy is desired, the surface of PDMS can be tailored by polydopamine (PDA), various polyethylene glycol (PEG) derivatives, or silane-based molecules. Hydrophobicity or hydrophilicity of PDMS surfaces can be easily rendered using one of these methods. There is a broad range of strategies and techniques to tailor PDMS properties in the literature which can be considered as one of the advantages of PDMS over other materials for microfabrication.
Tailoring the bulk properties of PDMS to improve its conductivity has become a topic of research in flexible and stretchable electronics. Different fillers such as carbon nanotubes, graphite, silver particles, nanowires, and gold nanotubes have been added to PDMS to reach higher conductivity. As PDA is conductive, it has been coated on PDMS to integrate electrochemical sensors for various applications.
With the origin of organ-on-a-chips and microfluidic cell culture platforms, PDMS has gained more attractions from researchers from other fields. As a result, the surface of PDMS has been coated with PDA, gelatine, collagen, or fibronectin to enhance the adhesion, proliferation, and the growth of cells inside a chip. Besides, the surface of PDMS first can be modified by PEG or a silane molecule or PDAas linker, and then a bioactive molecule can be attached to the linker for achieving specific applications such as differentiation or detection of a secreted biomolecule by cells.
PDMS is permeable to gases such as oxygen and carbon dioxide. This led to the origin of microfluidics blood oxygenators in which gas exchange between blood and air is needed.PDMS membranes can be easily fabricated by spin-coating wet PDMS on a flat substrate such as a wafer. The properties of the PDMS membrane can be tuned by changing the speed of the spinner, curing agent ratio, and curing temperature. PDMS membranes as thin as ~ 1 µm can be produced without observing pinhole defects. When the permeability should be avoided, the thickness of PDMS can be increased over 1 mm.
In a nutshell, PDMS has shown its potential in the realization of so many applications in microfluidics. Microfabrication with PDMS enables us to fabricate devices with simple designs to devices with very complex features. PDMS has been extensively studied, and this provides fantastic resources for everyone to optimize PDMS properties based on their needs.
Enjoyed this article? Don’t forget to share.
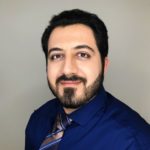
Mohammadhossein Dabaghi
Mohammadhossein Dabaghi is currently a postdoctoral fellow at the department of medicine at McMaster University. He completed his Ph.D. at McMaster University majoring in Biomedical Engineering and worked on new microfabrication technologies to develop an Artificial Placenta device for preterm neonates with respiratory failure. He earned a master’s degree in Biomedical/Chemical Engineering (2014), and a BS in Chemical Engineering from Sharif University of Technology, Iran (2012). His research interests focus on lung-on-a-chips, biosensors for organ-on-a-chips, and bioprinting.